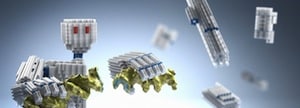
Several of our most recent posts on DNA origami and structural DNA nanotechnology have cited research aimed at creating more mechanically dynamic structures (here, here, and here). Another approach to using DNA origami to make nanomachines with moving parts has been published by a German research group inspired by how proteins function by forming and reconfiguring relatively weak bonds. A hat tip to ScienceDaily for reprinting this Technische Universitaet Muenchen news release published on AAAS EurekAlert “Designer’s toolkit for dynamic DNA nanomachines: Arm-waving nanorobot signals new flexibility in DNA origami“:
The latest DNA nanodevices created at the Technische Universitaet Muenchen (TUM) – including a robot with movable arms, a book that opens and closes, a switchable gear, and an actuator – may be intriguing in their own right, but that’s not the point. They demonstrate a breakthrough in the science of using DNA as a programmable building material for nanometer-scale structures and machines. Results published in the journal Science [abstract] reveal a new approach to joining – and reconfiguring – modular 3D building units, by snapping together complementary shapes instead of zipping together strings of base pairs. This not only opens the way for practical nanomachines with moving parts, but also offers a toolkit that makes it easier to program their self-assembly.
The field popularly known as “DNA origami,” in reference to the traditional Japanese art of paper folding, is advancing quickly toward practical applications, according to TUM Prof. Hendrik Dietz. Earlier this month, Dietz was awarded Germany’s most important research award, the Gottfried Wilhelm Leibniz Prize, for his role in this progress.
In recent years, Dietz and his team have been responsible for major steps in the direction of applications: experimental devices including a synthetic membrane channel made from DNA; discoveries that cut the time needed for self-assembly processes from a week to a few hours and enable yields approaching 100%; proof that extremely complex structures can be assembled, as designed, with subnanometer precision.
Yet all those advances employed “base-pairing” to determine how individual strands and assemblies of DNA would join up with others in solution. What’s new is the “glue.”
“Once you build a unit with base pairs,” Dietz explains, “it’s hard to break apart. So dynamic structures made using that approach tended to be structurally simple.” To enable a wider range of DNA nanomachines with moving parts and potentially useful capabilities, the team adapted two more techniques from nature’s biomolecular toolkit: the way proteins use shape complementarity to simplify docking with other molecules, and their tendency to form relatively weak bonds that can be readily broken when no longer needed.
Bio-inspired flexibility
For the experiments reported in Science, Dietz and his co-authors – doctoral candidates Thomas Gerling and Klaus Wagenbauer, and bachelor’s student Andrea Neuner from TUM’s Munich School of Engineering – took inspiration from a mechanism that allows nucleic acid molecules to bond through interactions weaker than base-pairing. In nature, weak bonds can be formed when the RNA-based enzyme RNase P “recognizes” so-called transfer RNA; the molecules are guided into close enough range, like docking spacecraft, by their complementary shapes.
The new technology from Dietz’s lab imitates this approach. To create a dynamic DNA nanomachine, the researchers begin by programming the self-assembly of 3D building blocks that are shaped to fit together. A weak, short-ranged binding mechanism called nucleobase stacking can then be activated to snap these units in place. Three different methods are available to control the shape and action of devices made in this way.
“What this has given us is a tiered hierarchy of interaction strengths,” Dietz says, “and the ability to position – precisely where we need them – stable domains that can recognize and interact with binding partners.” The team produced a series of DNA devices – ranging from micrometer-scale filaments that might prefigure technological “flagella” to nanoscale machines with moving parts – to demonstrate the possibilities and begin testing the limits.
For example, transmission electron micrographs of a three-dimensional, nanoscale humanoid robot confirm that the pieces fit together exactly as designed. In addition, they show how a simple control method – changing the concentration of positive ions in solution – can actively switch between different configurations: assembled or disassembled, with “arms” open wide or resting at the robot’s side.
Another method for switching a DNA nanodevice between its different structural states – by simply raising and lowering the temperature – proved to be especially robust. For earlier generations of devices, this required separating and re-joining DNA base pairs, and thus the systems were “worn out” by dilution and side-reactions after just a few cycles of switching. A scissor-like actuator described in the current paper underwent more than a thousand temperature-switched cycles over a four-day period with no signs of degradation.
“Temperature cycling is a way to put energy into the system,” Dietz adds, “so if the reversible conformational transition could be coupled to some continously evolving process, we basically now have a way not just to build nanomachines, but also to power them.”
“A snap” – like child’s play
There is yet another dimension to the flexibility gained by adding shape-complementary components and weak bonding to the DNA nanotechnology toolkit. Programming self-assembly by base-pairing alone is like writing computer code in machine language. The hope is that this new approach will make it easier to bend DNA origami toward practical ends, in much the same way the advent of higher-level computer programming languages spurred advances in software engineering.
Dietz compares it to building with children’s toys like LEGO: “You design the components to be complementary, and that’s it. No more fiddling with base-pair sequences to connect components.”
On his publications page, Prof. Dietz provides a full text PDF files of the Science paper. Also provided ia a “perspective” commentary by William Shih, published in the same issue of Science:
… Through their pioneering forays into shape complementarity, weak interfacial energies, and remotely tunable repulsion energies, Gerling et al. point the way toward reconfigurable, rigid DNA nanodevices that may one day rival the functional sophistication of the biomolecular machines of the cell.
This work and the work we cited earlier this year appear to present two different approaches to creating dynamic nanomachines. The earlier work focused on implementing well-defined motions, inspired by scaling macroscopic machines to the nanometer scale. This work focused on creating complementary shapes and modulating patterns of relatively weak interactions, inspired by protein biomolecular machinery. It should be really interesting to follow the progress of both lines of work to see what each brings to structural DNA nanotechnology and the molecular machine systems that it will make possible.
—James Lewis, PhD