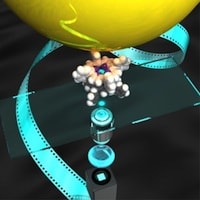
Molecular machines are a central component of efforts to develop atomically precise manufacturing. Optical microscopy and optical trap manipulation of single molecules, made possible by attachment of micrometer-scale beads, have facilitated greater understanding of the workings of biomolecular machines. For example, a 2008 paper published in Cell (“Intramolecular Strain Coordinates Kinesin Stepping Behavior along Microtubules“) revealed how the kinesin molecular motor molecule coordinates its two motor domains to achieve one-way stepping along microtubule proteins. When additional peptides were inserted into the mechanical “neck linker” elements that span the two motor domains, tension was reduced, and as a result, the motor’s velocity was reduced. Motor velocity returned to near normal when external tension was applied via an optical trap operating on a 920 nm diameter bead attached via an antibody to the molecular motor. Nanotechnologists can use similar techniques to study a wide variety of biomolecular machines, including naturally occurring molecular motors with typical length scales of 10 nm. Until now, however, it has not been possible to use similar approaches to study smaller synthetic molecular machines, with typical length scales on the order of one nm. A hat tip to Asian Scientist for reprinting this press release from the University of Tokyo “Seeing and touching a single 1-nm-sized synthetic molecular machine“:
Single-molecule imaging and manipulation with optical microscopy using a bead probe as a marker (single–molecule “motion capturing”) unveils fundamental properties of biomolecular machines such as direction of motion, step size and force the molecule exerts, which cannot be resolved by whole-molecule measurements. As a result, it has become an essential method for research of biomolecular machines. In addition, single-molecule motion capturing could also become a powerful tool to develop “synthetic” molecular machines. However, it is difficult to apply the conventional method to individual molecules because the size of a typical synthetic molecular machine is only 1 nm, about one-tenth the size of a biomolecular machine. This miniaturization of the target molecule causes significant problems such as low efficiency of the bead probe immobilization reaction and undesired interaction between the surfaces of the bead and substrate.
[University of Tokyo researchers] captured the motion of a single synthetic machine about 1 nm in size for the first time. In this experiment, the researchers resolved the problems in the conventional method which are caused by the small size of the target and successfully visualized the rotational motion of a single double-decker porphyrin (DD), known as a synthetic molecular bearing, by imaging a bead on DD. Furthermore the researchers successfully manipulated the motion of the single DD molecule by applying an external force to the bead.
This method, that allows us to “see and touch” single synthetic molecular machines, provides currently the only strategy to verify and evaluate the performance of synthetic molecular motors generating force, one of the ultimate goals in the development of synthetic molecular machines. For example, if it were possible to create a light-driven synthetic molecular motor connected to a biomolecular motor, it should then be possible to establish a tailor-made energy conversion system that can control various chemical reactions by application of light. Therefore this seminal technique will contribute to establishment of tailor-made energy conversion systems based on molecular machines.
This research has been featured on the back cover of the journal Angewandte Chemie International Edition [abstract, full text PDF courtesy of senior author].
The 1-nm sized double-decker porphyrin (DD) is based on a meso-tetraaryl DD. The potential barrier for rotation of a DD depends on the bulkiness of the substituents on the porphyrin ring side chains and on the ionic radius of the central metal ion, a cerium ion (CeIII). Substituents included terminal azide groups to enable click chemistry for linking to an alkyne-modified magnetic bead or glass substrate. The rotational dynamics of the 200-nm magnetic bead were visualized using optical microscopy, revealing rotation only in discrete steps of 90° each, as expected from the symmetry of the DD. The motions visualized are Brownian and passive. It would be interesting to see similar results from the application of force by a molecular machine.
—James Lewis, PhD