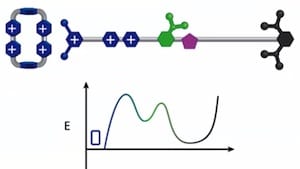
Among the classes of molecular machines that make life possible are the protein pumps that move ions and small molecules across membranes, from one compartment to another. These ions and molecules are driven uphill energetically, from low concentrations to high concentrations, forming systems that are high in energy and can perform work on their environments. Demonstration of this basic principle using a much smaller artificial molecular pump comes this month from the laboratory of Sir Fraser Stoddart, winner of the 2007 Feynman Prize in Nanotechnology in the Experimental category. Hat tips to Phys.org and ECN for reprinting this Northwestern University news release written by Megan Fellman “Nature inspires first artificial molecular pump“:
Using nature for inspiration, a team of Northwestern University scientists is the first to develop an entirely artificial molecular pump, in which molecules pump other molecules. This tiny machine is no small feat. The pump one day might be used to power other molecular machines, such as artificial muscles.
The new machine mimics the pumping mechanism of life-sustaining proteins that move small molecules around living cells to metabolize and store energy from food. For its food, the artificial pump draws power from chemical reactions, driving molecules step-by-step from a low-energy state to a high-energy state — far away from equilibrium.
While nature has had billions of years to perfect its complex molecular machinery, modern science is only beginning to scratch the surface of what might be possible in tomorrow’s world.
“Our molecular pump is radical chemistry — an ingenious way of transferring energy from molecule to molecule, the way nature does,” said Sir Fraser Stoddart, the senior author of the study. Stoddart is the Board of Trustees Professor of Chemistry in Northwestern’s Weinberg College of Arts and Sciences.
“All living organisms, including humans, must continuously transport and redistribute molecules around their cells, using vital carrier proteins,” he said. “We are trying to recreate the actions of these proteins using relatively simple small molecules we make in the laboratory.”
Details of the artificial molecular pump were published May 18 by the journal Nature Nanotechnology [abstract].
Chuyang Cheng, a fourth-year graduate student in Stoddart’s laboratory and first author of the paper, has spent his Ph.D. studies researching molecules that mimic nature’s biochemical machinery. He first designed an artificial pump two years ago, but it required more than a year of testing prototypes before he found the ideal chemical structure.
“In some respects, we are asking the molecules to behave in a way that they would not do normally,” Cheng said. “It is much like trying to push two magnets together. The ring-shaped molecules we work with repel one another under normal circumstances. The artificial pump is able to syphon off some of the energy that changes hands during a chemical reaction and uses it to push the rings together.”
The tiny molecular machine threads the rings around a nanoscopic chain — a sort of axle — and squeezes the rings together, with only a few nanometers separating them. At present, the artificial molecular pump is able to force only two rings together, but the researchers believe it won’t be long before they can extend its operation to tens of rings and store more energy.
Stoddart’s team has been researching artificial molecular machines for several years. A challenge they have faced for a long time is how to power their machines. This latest advance may allow them to make machines that perform tasks at the molecular level.
Compared to nature’s system, the artificial pump is very simple, but it is a start, the researchers say. They have designed a novel system, using kinetic barriers, that allows molecules to flow “uphill” energetically.
“This is non-equilibrium chemistry, moving molecules far away from their minimum energy state, which is essential to life,” said Paul R. McGonigal, an author of the study. “Conducting non-equilibrium chemistry in this way, with simple artificial molecules, is one of the major challenges for science in the 21st century.”
Ultimately, they intend to use the energy stored in their pump to power artificial muscles and other molecular machines. The researchers also hope their design will inspire other chemists working in non-equilibrium chemistry.
“This is completely unlike the process of designing the machinery we are used to seeing in everyday life,” Stoddart said. “In a way, one must learn to see things from the molecules’ point of view, considering forces such as random thermal motion that one would never consider when building an agricultural water pump or any other mechanical device.”
In this work rapid cycling between a reducing and an oxidizing solution environment achieved by adding either a reducing chemical or an oxidizing chemical to the system resulted in concentrating two cationic ring molecules on an oligomethylene chain making up one end of a rotaxane molecule. Although the redox cycling occurs in a few minutes, the precisely organized non-covalent binding interactions that form the kinetic barrier to moving backward take a couple hours to occur. The net result is that redox energy has been stored in one molecular compartment that could in principle be used to perform work. The authors believe that they should be able to store a few tens of such molecules on a similar rotaxane with a longer oligomethylene chain. The next question beyond how much energy can they store is, what mechanisms can they devise to use that energy to perform molecular work. For example, could the energy be used to make or break covalent bonds, thus perhaps forming a component for an atomically precise manufacturing system?
—James Lewis, PhD