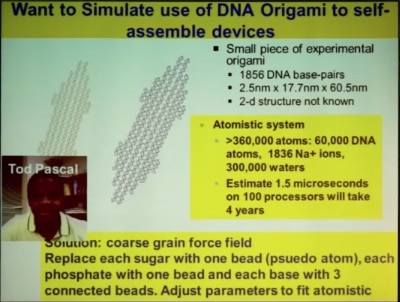
A select set of videos from the 2013 Foresight Technical Conference: Illuminating Atomic Precision, held January 11-13, 2013 in Palo Alto, have been made available on vimeo. Videos have been posted of those presentations for which the speakers have consented. Other presentations contained confidential information and will not be posted.
The seventh speaker at the Computation and Molecular Nanotechnolgies session, William Goddard, presented “Nanoscale Materials, Devices, and Processing Predicted from First Principals”. https://vimeo.com/62119945 – video length 34:22. Prof. Goddard addressed some of the method developments to allow modeling of large-scale systems, followed by some examples. He noted that the grand vision over the past 25 years has been that theory can be used to predict something useful. To predict new systems where there is no empirical data it is necessary to start with first principles.
Prof Goddard reviewed the advances that enabled going from first principles to nanoscopic systems of interest. Starting from quantum mechanics to describe a few hundred—perhaps a thousand—atoms, it was necessary to describe realistic temperatures, pressures, and concentrations in systems with millions or billions of atoms.
In describing work in his group, Prof Goddard noted that 40% of their efforts were spent developing new methods to solve problems, but they get only 5% of their funding for doing that because people want answers to problems, not new methods.
Prof Goddard proceeded to discuss four of the breakthroughs that they have made in addressing these issus: (1) the eFF approach of doing non adiabatic quantum mechanics on systems of highly excited electrons; (2) the Reax approach for doing reactions on millions of atoms, with good descriptions of barriers; (3) a fast way of doing entropy in the same time scale used for electrodynamics, free energy; (4) describing the coarse-grained force fields for DNA and RNA nanotechnology to try to assemble devices.
Prof. Goddard continued that there are four applications of interest for the eFF approach:
- model processes involved in damage-free low-energy electron enhanced etching
- what happens when electrons are ejected from cracked semiconductors like silicon
- look at what happens when a field is applied and electrons come through and cause excitations leading to dielectric breakdown
- simulate the process of an STM forming images based on the structure of the tip and the surface
The issue in eFF, Prof. Goddard continued, is that electrons are in highly excited states, hopping off perhaps with plasma nearby—not the first or second excited state but perhaps the millionth excited state. The approach taken is to simplify the electrons, describing them as Gaussian functions, the normal electrostatic interactions between electrons and nuclei and of electrons with each other are evolved. One serious approximation is made by taking the wave function as a Hartree product (multiplying together the million orbitals of a million electrons), and then propagate this wave function with Hamiltonians. The Pauli principle is introduced by recognizing that the Pauli principle forces two orbitals with the same spin to be orthogonal to each other. Figuring out what kind of force orthogonalizing two Gaussians leads to results in obtaining three scaling parameters, all near 1, obtained from calculations on small systems.
One of the problems Prof Goddard’s group applied this procedure to was to understand what happens with low-energy electron enhanced etching od semiconductors to get the incredibly smooth surfaces that are seen. They speculated that maybe this is an Auger effect, that maybe what the electrons do is excite a silicon core electron, and when that vacancy is filled there is enough energy to kick out a second electron, breaking the bond. They used the eFF process to simulated the Auger process to find out what the electrons are actually doing. They ionized the down-spin carbon 1s electron in a diamondoid with a couple hundred atoms, four electrons in the four bonds to that atom rush in to try to fill the hole. The Auger electron is kicked out, and in the particular case Prof Goddard presented, and CH radical is also kicked out. Repeating the simulation many times identifies all of the possible reactions that can happen. One result was that all of the carbon atoms that get etched away come from the surface, explaining why this process leads to smooth surfaces with no damage. Prof Goddard noted that no other quantum mechanics-based method can handle Auger decay processes, and that eFF can handle highly excited electronic states. Current efforts in this area include looking at whether electrons can be tuned to have differential effects even without masks on the surface.
Another eFF success that Prof Goddard notes is simulating what happens when silicon fractures. It has been experimentally observed that electrons will leave the system, producing current flow and positive and negative voltage fluctuations as the crack propagates through the silicon. The eFF approach predicts the experimentally observed propagation rate, which has not been achieved by any classical approach.
The second breakthrough that Prof Goddard presented is their ReaxFF reactive force field as a solution for the problem that even simple nanodevices to be modeled, such as something 25x54x27 nm, contains 3.7 million atoms, which is too many to be practical for any quantum mechanics (QM) based method. However, their reactive force field describes the atoms almost as well as does QM, and 3.7 million atoms is a practical size. In the ReaxFF system charges are allowed to flow in the system, just as in QM, as bonds break and form. The bonds are aso allowed to change bond order and energy as the bond stretches. All the parameters come from QM. There is no dependence on empirical data, which is important with these materials–based systems because there is almost never enough empirical data to determine a system. With ReaxFF, Prof Goddard continued, one force field can describe vanadium metal, vanadium oxide,and vanadium oxidation states II, III, IV and V. The example he presented had to do with the successful prediction of hot spot formation in a material segment of 3.7 million atoms.
The third breakthrough that Prof Goddard presented addresses the difficulty of getting free energies. Usually talk of energies is restricted to enthalpies, or in the case of QM, enthalpies at 0 K. We want, Prof Goddard continued, to be able to calculate at the appropriate temperature and pressure and get free energies. THe method they developed has the same costs as doing dynamics, while previous methods for doing entropies were about 3000 times slower. One example looking at a branched chain DNA structure of about 50,000 atoms to determine how free energy, enthalpy, and entropy change over about 100 ns revealed that this process is dominated by entropy—a hydrophobic effect of release of water from the system into the surrounding solvent shell that dominates the system. Entropy calculations are fundamentally an equilibrium situation, but all that is needed is an equilibrium calculation of 10 to 20 ps. To do the calculation a density of states was obtained from molecular dynamics calculations, which was used to calculate partition function and entropy, etc.
The fourth breakthrough that Prof Goddard presented addressed the DNA origami technique: using DNA to organize large systems. A typical problem could involve 1856 DNA base-pairs and a structure 2.5×17.7×60.5 nm involving more than 360,000 atoms including counterions and water molecules. THe estimated time to complete a simulation of 1.5 µs using 100 processors would be about 4 years. Their solution was to invent a coarse-grained force field in which each sugar moiety is replaced with one bead (pseudo atom), each phosphate with one bead, and each base with three connected beads. In collaboration with DNA origami experimentalists, Prof Goddard’s group simulated using DNA origami to assemble structures of carbon nanotubes. They designed sequences for the linker and DNA hooks to ensure that various assumptions made to design the nanostructures were realistic. They worked out conditions to deposit the carbon nanotubes on either face of the origami structure and for depositing the nanostructures on silicon dioxide and for positioning various nanoparticles on the DNA origami.
—James Lewis, PhD