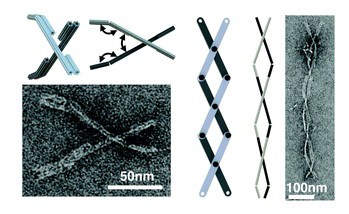
Recently we pointed to work at Ohio State University that demonstrated programmed complex motions in simple molecular machines fabricated using scaffolded DNA origami. This accomplishment was the fruit of their systematic effort to implement macroscale engineering design principles in DNA molecular machinery. This past month they published a review of their approach “Mechanical design of DNA nanostructures” in the Royal Society of Chemistry journal Nanoscale. The abstract:
Structural DNA nanotechnology is a rapidly emerging field that has demonstrated great potential for applications such as single molecule sensing, drug delivery, and templating molecular components. As the applications of DNA nanotechnology expand, a consideration of their mechanical behavior is becoming essential to understand how these structures will respond to physical interactions. This review considers three major avenues of recent progress in this area: (1) measuring and designing mechanical properties of DNA nanostructures, (2) designing complex nanostructures based on imposed mechanical stresses, and (3) designing and controlling structurally dynamic nanostructures. This work has laid the foundation for mechanically active nanomachines that can generate, transmit, and respond to physical cues in molecular systems.
In reviewing efforts to understand mechanical behavior of DNA nanostructures, this 9-page review provides a very good bird’s eye view of 33 years of DNA nanotechnology research. The first area to receive focused attention is the design and characterization of mechanical bending stiffness in DNA origami nanostructures. A large body of work establishes that bundling a number of dsDNA helices into compact bundles can produce several orders of magnitude increase in bending stiffness, approaching the stiffness of protein bundles made of actin or microtubules. A combination of simulations and experiments demonstrate that nanostructures with a cross-section of 18 or more dsDNA helices are not subject to significant thermal fluctuations. These results and the computational tools developed to obtain them are expected to enable the design of devices to withstand and transmit forces through interaction with other materials and molecules.
The incorporation of designed stresses into DNA nanostructures has already been used to introduce twist and curvatures into designs and to store energy in stressed structures. Entropic elasticity of single=stranded DNA can be balanced with compression or bending of dsDNA components to control both stiffness and geometry. The point is made that ultimately the mechanical stress that can be introduced or the local force that can be applied must be less than the base pairing energy of the nanostructure. However, the authors point out that design modification like incorporating more stable components (such as peptide nucleic acids) or chemical modifications (such as covalent cross-links between strands) “could allow for mechanically enhanced structures that can withstand and transmit larger forces or store more mechanical energy.” For those who look to structural DNA nanotechnology as a road toward atomically precise manufacturing, these suggestions raise interesting questions. Can DNA nanostructures, or composities built upon DNA nanostructures be made stiff enough and made to transmit sufficient force to make or break covalent bonds?
The third and final section on designing and actuating dynamic behavior culminates with the authors’ efforts to adapt macroscopic engineering machine design approaches to scaffolded DNA origami, starting with fabricating joints with constrained motions in specific rotational or translational degrees of freedom.They have made several of these DNA origami mechanisms (DOM) that produce either controlled angular motion or controlled linear motion. The next step was producing more complex motion by incorporating multiple joints into a single structure. Their recently published crank-slider can convert rotational motion generated by a binding event into linear motion to generate a linear force, or it could generate a rotational torque in response to a linear displacement. Included here are two hitherto unpublished machine components: a universal joint and a scissors mechanism that transforms rotation about three joints into linear extension, resembling a macroscopic scissors lift. The authors note that in this case the motion was achieved by thermal fluctuation, but they intend in future work to couple distributed molecular actuation to force generation and micrometer scale motion. The authors conclude:
With the ability to design and control complex mechanical behavior, combined with chemically functionalizing DNA nanostructures and recent advances towards fabricating larger DNA origami systems… it seems the functional scope of DNA nanotechnology will continue to expand for years to come.
Sounds right to me!
—James Lewis, PhD