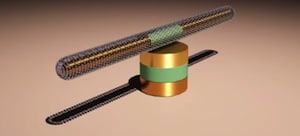
In a post here a number of years ago then-Foresight President J. Storrs Hall commented on the power density that nanomotors based on advanced nanotechnology are expected to have—on the order of a megawatt in a cubic millimeter. How is current research in nanomotors progressing? Last year Phys.Org reprinted this University of Texas at Austin news release “Engineers Build World’s Smallest, Fastest Nanomotor“:
Researchers at the Cockrell School of Engineering at The University of Texas at Austin have built the smallest, fastest and longest-running tiny synthetic motor to date. The team’s nanomotor is an important step toward developing miniature machines that could one day move through the body to administer insulin for diabetics when needed, or target and treat cancer cells without harming good cells.
With the goal of powering these yet-to-be invented devices, UT Austin engineers focused on building a reliable, ultra-high-speed nanomotor that can convert electrical energy into mechanical motion on a scale 500 times smaller than a grain of salt.
Mechanical engineering assistant professor Donglei “Emma” Fan led a team of researchers in the successful design, assembly and testing of a high-performing nanomotor in a nonbiological setting. The team’s three-part nanomotor can rapidly mix and pump biochemicals and move through liquids, which is important for future applications. The team’s study was published in the April [2014] issue of Nature Communications [abstract].
Fan and her team are the first to achieve the extremely difficult goal of designing a nanomotor with large driving power.
With all its dimensions under 1 micrometer in size, the nanomotor could fit inside a human cell and is capable of rotating for 15 continuous hours at a speed of 18,000 RPMs, the speed of a motor in a jet airplane engine. Comparable nanomotors run significantly more slowly, from 14 RPMs to 500 RPMs, and have only rotated for a few seconds up to a few minutes.
Looking forward, nanomotors could advance the field of nanoelectromechanical systems (NEMS), an area focused on developing miniature machines that are more energy efficient and less expensive to produce. In the near future, the Cockrell School researchers believe their nanomotors could provide a new approach to controlled biochemical drug delivery to live cells.
To test its ability to release drugs, the researchers coated the nanomotor’s surface with biochemicals and initiated spinning. They found that the faster the nanomotor rotated, the faster it released the drugs.
“We were able to establish and control the molecule release rate by mechanical rotation, which means our nanomotor is the first of its kind for controlling the release of drugs from the surface of nanoparticles,” Fan said. “We believe it will help advance the study of drug delivery and cell-to-cell communications.”
The researchers address two major issues for nanomotors so far: assembly and controls. The team built and operated the nanomotor using a patent-pending technique that Fan invented while studying at Johns Hopkins University. The technique relies on AC and DC electric fields to assemble the nanomotor’s parts one by one.
In experiments, the researchers used the technique to turn the nanomotors on and off and propel the rotation either clockwise or counterclockwise. The researchers found that they could position the nanomotors in a pattern and move them in a synchronized fashion, which makes them more powerful and gives them more flexibility.
Fan and her team plan to develop new mechanical controls and chemical sensing that can be integrated into nanoelectromechanical devices. But first they plan to test their nanomotors near a live cell, which will allow Fan to measure how they deliver molecules in a controlled fashion.
The procedure used to assemble these nanomotors is fast and simple using electric tweezers they previously described, which can transport nanowires and nanotubes along arbitrary trajectories to designated positions with a precision of 150 nm. Three-segment gold/nickel/gold nanowires (150 to 400 nm in diameter and 800 nm to 10 µm in length) serve as rotors and are fabricated by electrodeposition in templates. The short nickel segment anchors the nanowire rotor to the magnetic bearing (200 nm to 2 µm in diameter thin film stacks of gold, nickel, and chromium). The nanowires can be transported by the DC electric field and aligned either parallel or perpendicular to their transport direction by the AC electric field. By controlling the durations of the combined AC and DC field in the X and Y directions, the nanowires can be manipulated in two dimensions. When positioned near the magnetic bearing, they will snap into position atop the bearing. The authors state that with a little practice a K-12 student was able to assemble a nanomotor in about 10 seconds.
Once anchored on the bearing, the electric field will no longer move the rotor, but will cause it to rotate at a speed and direction determined by the rotating electric field. Quadrupole microelectrodes rotate the assembled nanomotors with prescribed angle, speed and direction according to the four AC voltages applied to the four sub-electrodes. Rotation of the rotors immediately starts and stops with changes in the field because of the very high viscosity caused by the very low Reynolds number of nanowires in water. A detailed mathematical model of the nanoscale forces operating on the nanomotors was constructed and validated to predict how the motors will behave under various conditions. The authors do not estimate the energy density attained by their nanomotors, but certainly a speed of 18000 r.p.m. is not shabby. In any case, power densities of the magnitude to be provided in the future by atomically precise manufacturing will not be needed to do interesting things with NEMS, microfluidics and lab-on-a-chip applications.
—James Lewis, PhD