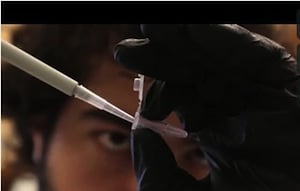
Part of the problem in building complex molecular machine systems—whether the evolved systems that are the foundation of biology, or the artificial systems being designed to implement various nanotechnology applications eventually leading to high-throughput atomically precise manufacturing (APM)—is the fabrication and organization of the needed nanostructures. The other part is understanding how these molecular components interact so that these interactions can be orchestrated to accomplish the desired functions. Conventional methods for studying molecular interactions are difficult and expensive, but now a simple form of DNA nanotechnology provides a method to make such studies much more accessible, as described in this press release from the Wyss Institutue “DNA nanoswitches reveal how life’s molecules connect“:
An accessible new way to study molecular interactions could lower cost and time associated with discovering new drugs
A complex interplay of molecular components governs almost all aspects of biological sciences — healthy organism development, disease progression, and drug efficacy are all dependent on the way life’s molecules interact in the body. Understanding these bio–molecular interactions is critical for the discovery of new, more effective therapeutics and diagnostics to treat cancer and other diseases, but currently requires scientists to have access to expensive and elaborate laboratory equipment.
Now, a new approach developed by researchers at the Wyss Institute for Biologically Inspired Engineering, Boston Children’s Hospital and Harvard Medical School promises a much faster and more affordable way to examine bio–molecular behavior, opening the door for scientists in virtually any laboratory world–wide to join the quest for creating better drugs. The findings are published in February’s issue of Nature Methods. [full text PDF, protocol, videos, author file courtesy of senior author]
“Bio–molecular interaction analysis, a cornerstone of biomedical research, is traditionally accomplished using equipment that can cost hundreds of thousands of dollars,” said Wyss Associate Faculty member Wesley P. Wong, Ph.D., senior author of study. “Rather than develop a new instrument, we’ve created a nanoscale tool made from strands of DNA that can detect and report how molecules behave, enabling biological measurements to be made by almost anyone, using only common and inexpensive laboratory reagents.”
Wong, who is also Assistant Professor at Harvard Medical School in the Departments of Biological Chemistry & Molecular Pharmacology and Pediatrics and Investigator at the Program in Cellular and Molecular Medicine at Boston Children’s Hospital, calls the new tools DNA “nanoswitches”.
Nanoswitches comprise strands of DNA onto which molecules of interest can be strategically attached at various locations along the strand. Interactions between these molecules, such as successful binding of a drug compound with its intended target, such as a protein receptor on a cancer cell, cause the shape of the DNA strand to change from an open and linear shape to a closed loop. Wong and his team can easily separate and measure the ratio of open DNA nanoswitches vs. their closed counterparts through gel electrophoresis, a simple lab procedure already in use in most laboratories, that uses electrical currents to push DNA strands through small pores in a gel, sorting them based on their shape.
“Our DNA nanoswitches dramatically lower barriers to making traditionally complex measurements,” said co–first author Ken Halvorsen, formerly of the Wyss Institute and currently a scientist at the RNA Institute at University of Albany. “All of these supplies are commonly available and the experiments can be performed for pennies per sample, which is a staggering comparison to the cost of conventional equipment used to test bio–molecular interactions.”
To encourage adoption of this method, Wong and his team are offering free materials to colleagues who would like to try using their DNA nanoswitches.
“We’ve not only created starter kits but have outlined a step–by–step protocol to allow others to immediately implement this method for research in their own labs, or classrooms,” said co–first author Mounir Koussa, a Ph.D. candidate in neurobiology at Harvard Medical School.
“Wesley and his team are committed to making an impact on the way bio–molecular research is done at a fundamental level, as is evidenced by their efforts to make this technology accessible to labs everywhere,” said Wyss Institute Founding Director Donald Ingber, M.D., Ph.D., who is also the Judah Folkman Professor of Vascular Biology at Boston Children’s Hospital and Harvard Medical School and a Professor of Bioengineering at Harvard SEAS. “Biomedical researchers all over the world can start using this new method right away to investigate how biological compounds interact with their targets, using commonly–available supplies at very low cost.”
Anyone interested in learning more about how to use DNA nanoswitches in their lab can watch a protocol video series and request free materials for making them at wyss.harvard.edu/nanoswitch.
For a nanoswitch scaffold upon which to attach the interacting molecules, this method uses the same 7249-nucleotide ssDNA from M13 phage used for scaffolded DNA origami. The large size of the DNA allows incorporating thousands of dye molecules to make the technique very sensitive. The interacting molecules are positioned on the scaffold using 60-nucleotide short strands such that interaction of the attached biomolecules causes the DNA to form a loop that can be resolved from linear molecules by a simple gel electrophoresis assay. Letting the attached molecules interact under various conditions for varying lengths of time with or without the presence of competing molecules, yields a large number of parameters of the interactions. The method was extensively characterized using the very well studied biotin-streptavidin interaction. The method was able to measure rates for processes occurring over a time scale of seconds to months. The method was demonstrated to measure molecular interactions over a large size range, from large proteins with molecular weights of 150,000 to the dissociation of two sulfur atoms upon the reduction of a disulfide bond. It was even possible to study a complex four-body interaction with five discernable states, a feat difficult or impossible to achieve using conventional methods.
The ability to quickly and inexpensively measure interactions of such complexity will no doubt be immediately useful to many researchers working in various branches of biotechnology. How long will it take for work with artificial molecular machine systems to reach the point of being able to benefit from such capability?
—James Lewis, PhD