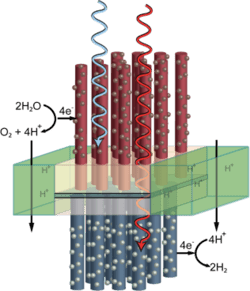
A few weeks ago Science published a review written by California Institute of Technology Chemistry Professor Nate Lewis titled “Research opportunities to advance solar energy utilization”. The link to the paper from Lewis’s publication page leads to the full text article on the journal’s web site. Prof. Lewis’s one-page summary of his own review concludes that “Considerable opportunities for cost reduction that can achieve both evolutionary and revolutionary performance improvements are present for all types of solar energy–conversion technologies.” The review enumerates those technologies, focusing on production of solar electricity through photovoltaics, solar thermal, and artificial photosynthesis to produce fuels from sunlight. Two fundamental constraints on solar energy systems are made explicit: (1) the low energy density of sunlight at Earth’s surface, necessitating large areas needed to capture and convert solar power; (2) the intermittent nature of sunlight, requiring affordable technologies for large-scale energy storage.
The first indication of the variety of opportunities available is the table listing a dozen types of photovoltaic materials available to absorb light and produce electricity, characterized according to the nature of the material, the maturity of the technology, production, and efficiency. The materials listed vary greatly in terms of chemical nature, physical form, and how they are currently manufactured. Increasing control over the structure of matter, ultimately approaching atomic precision, will give greater control over ease of manufacture, cost, durability, and efficiency of energy conversion. In addition to the efficiency of energy capture and conversion, factors like single crystal or thin film, or how well the photovoltaic material is encapsulated can affect, weight, ease and cost of installation, keeping toxic materials out of the environment, etc. Opportunities to improve current and near-term systems through research and innovation are accordingly more varied than immediately apparent. Another opportunity is cost reduction through replacement of expensive materials with earth-abundant materials.
As an isolated example of how opportunities for reduced cost and increased convenience lurk in non-obvious places, Lewis gives the example of heavy glass required to protect solar panels from hailstorms, causing solar panels to be inflexible and difficult to install and maintain, plus leading to increased costs for shipping, installation labor, and structural support. Atomically precise materials with much greater strength to weight ratios than conventional materials would likely find application here.
Lewis notes “The cost of persistent grid-scale storage currently far exceeds the levelized cost of solar electricity,” leading to a discussion of costs and research opportunities for improving battery storage.
The section on solar thermal power describes that different types of solar collecting systems working at temperatures ranging from 550 to 1200°C and located in areas of intense sunshine, such as the US southwest or Australia or north Africa, are inspiring research in areas like fabrication of materials that allow operation at higher temperatures.
A final section of the review describes research progress in the direct production of fuels using sunlight, such as artificial photosynthesis based on photoelectrochemical cells.
I will not try to describe the research opportunities involving various forms of nanotechnology pointed to in this review, but as an indication of the breadth of on-going work in this area, the following seven energy-related posts appeared over at Nanowerk during the past two days:
Refined protective layer for the ‘artificial leaf’
A process for providing sensitive semiconductors for solar water splitting (‘artificial leaves’) with an organic, transparent protective layer has been developed by researchers. The extremely thin protective layer made of carbon chains is stable, conductive, and covered with catalyzing nanoparticles of metal oxides. These accelerate the splitting of water when irradiated by light.Solar water splitting using particulate photocatalyst sheets
Researchers at the University of Tokyo have achieved a solar-to-hydrogen energy conversion efficiency of 1.1% using particulate photocatalyst sheets developed by the research group. The photocatalyst sheet can potentially produce a large quantity of solar hydrogen inexpensively owing to its scalability and low fabrication cost.Solar cells: Silicon profits from a dose of iron
By rapidly heating silicon wafers covered with thin iron silicide and aluminum films, A*STAR researchers have developed a way to eliminate many of the complicated, time-consuming steps needed to fabricate light harvesting solar cells.Solid electrolytes open doors to solid-state batteries
Japanese scientists have synthesized two crystal materials that show great promise as solid electrolytes. All-solid-state batteries built using the solid electrolytes exhibit excellent properties, including high power and high energy densities, and could be used in long-distance electric vehicles.Scientists extend the reach of single crystals
Materials scientists and physicists at Lehigh University have demonstrated a new method of making single crystals that could enable a wider range of materials to be used in microelectronics, solar energy devices and other high-technology applications.Pumping up energy storage with metal oxides and graphene
Material scientists at Lawrence Livermore National Laboratory have found certain metal oxides increase capacity and improve cycling performance in lithium-ion batteries. The team synthesized and compared the electrochemical performance of three graphene metal oxide nanocomposites and found that two of them greatly improved reversible lithium storage capacity.Carbon leads the way in clean energy
Groundbreaking research at Griffith University is leading the way in clean energy, with the use of carbon as a way to deliver energy using hydrogen. Professor Xiangdong Yao and his team from Griffith’s Queensland Micro- and Nanotechnology Centre have successfully managed to use the element to produce hydrogen from water as a replacement for the much more costly platinum.
The technologies that are relevant to energy applications range from nanomaterials and nanoscale processing to complex atomically precise arrangements of catalysts. It will be interesting to see how the mix evolves as technology improves and provides more effective solutions at lower costs.
—James Lewis, PhD