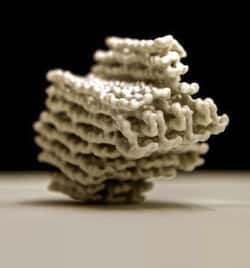
Before this year the best way to build complex 3D nanostructures from DNA was to use scaffolded DNA origami (see, for example, this post). Last May scientists at the Wyss Institute introduced a DNA tile method for fabricating complex DNA objects that was much faster and much less expensive, and just two weeks ago we posted news that they had extended this method to make arbitrarily complex 3D DNA nanostructures from DNA bricks. Now scientists at the Technische Universität München have published two papers documenting major enhancements to scaffolded DNA origami. From “Reality check for DNA nanotechnology“:
Two major barriers to the advancement of DNA nanotechnology beyond the research lab have been knocked down. This emerging technology employs DNA as a programmable building material for self-assembled, nanometer-scale structures. Many practical applications have been envisioned, and researchers recently demonstrated a synthetic membrane channel made from DNA. Until now, however, design processes were hobbled by a lack of structural feedback. Assembly was slow and often of poor quality. Now researchers led by Prof. Hendrik Dietz of the Technische Universitaet Muenchen (TUM) have removed these obstacles.
One barrier holding the field back was an unproven assumption. Researchers were able to design a wide variety of discrete objects and specify exactly how DNA strands should zip together and fold into the desired shapes. They could show that the resulting nanostructures closely matched the designs. Still lacking, though, was the validation of the assumed subnanometer-scale precise positional control. This has been confirmed for the first time through analysis of a test object designed specifically for the purpose. A technical breakthrough based on advances in fundamental understanding, this demonstration has provided a crucial reality check for DNA nanotechnology.
In a separate set of experiments, the researchers discovered that the time it takes to make a batch of complex DNA-based objects can be cut from a week to a matter of minutes, and that the yield can be nearly 100%. They showed for the first time that at a constant temperature, hundreds of DNA strands can fold cooperatively to form an object — correctly, as designed — within minutes. Surprisingly, they say, the process is similar to protein folding, despite significant chemical and structural differences. “Seeing this combination of rapid folding and high yield,” Dietz says, “we have a stronger sense than ever that DNA nanotechnology could lead to a new kind of manufacturing, with a commercial, even industrial future.” And there are immediate benefits, he adds: “Now we don’t have to wait a week for feedback on an experimental design, and multi-step assembly processes have suddenly become so much more practical.” …
To test the unproven assumption of subnanometer-scale precise positional control, the TUM scientists and their collaborators at MRC Laboratory of Molecular Biology in Cambridge, UK built a large asymmetrical 3D DNA nanostructure incorporating distinctive design motifs, and then characterized its structure with low-temperature electron microscopy. The research was published recently in PNAS (abstract, open access PDF). They designed a DNA nanostructure comprising 15,328 nucleotides (more than 460,000 atoms) assembled from a 7,249-nucleotide long scaffold strand of bacteriophage DNA and 163 short staple strands. The structure formed overnight in a one-pot reaction in high yield. Cryo-electron microscopy enabled a 3D reconstruction based upon tens of thousands of individual images. The resolution of the reconstructed image was sub-nanometer but not quite atomically precise, ranging from 0.97 nm in the core of the nanostructure to 1.4 nm at the periphery. Analysis of the structure determined indicates that the structural order within the nanostructure is comparable to that of natural nanomachines. Detailed comparison of the obtained structure with the designed structure showed more variation than expected in the structure of the DNA helices formed, indicating that the densely packed design led to some unusual DNA topologies. These results indicate that an interactive strategy of designing a folded DNA structure followed by 3D structural analysis will allow construction of a rich variety of precise, complex objects. The authors conclude:
By using chemical groups attached to DNA strands or even reactive motifs formed by DNA itself, this strategy offers an attractive route to achieving complex functionalities known today only from natural nanomachines.
In a second paper just published in Science [abstract], the TUM researchers tackle a major limitation of scaffolded DNA origami: week-long reaction times as the mixture of template and staple DNA strands is very slowly cooled over a very large temperature range, and poor yields. The researchers very carefully followed the rate of structure formation for three different DNA nanostructures as reaction mixes were slowly cooled over a very broad temperature range. One DNA nanostructure was a multilayer platelike structure, one a bricklike object, and a third a gearlike object. They found that the DNA nanostructures each formed at a very narrow temperature range (of about 4° C) that was different for each DNA nanostructure. In addition, the folding was complete in as little as 15 minutes. By choosing the appropriate temperature for each DNA nanostructure, the folding could be complete at constant temperature in as little as 5 minutes. Further, folding at constant temperature greatly increased the yield of correctly folded nanostructures. For several different nanostructures, the increase in yield compared to previous protocols ranged from 7-fold to 330-fold improvement. In absolute terms, the yield of properly folded nanostructres approached 100%. The authors note that several attributes of the folding they observe with their protocols resemble the folding of proteins, despite the chemical and structural differences between proteins and DNA.
From the standpoint of DNA nanotechnology as a component of the Technology Roadmap for Productive Nanosystems, the high yield of well-folded building blocks opens the door to hierarchical assembly of larger objects. It will also greatly facilitate the process of fine-tuning the design of functional molecular machine systems incorporating complex DNA nanostructures. The respective roles to be played by DNA bricks and scaffolded DNA origami, of course, remain to be seen.
—James Lewis, PhD