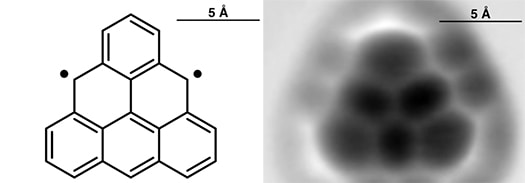
The application of scanning probe microscopy to building individual molecules on a surface took another step forward with the fabrication of a fragment of graphene that was too reactive to be synthesized using conventional chemistry. Over at Quartz Akshat Rathi describes how “IBM researchers have created an ‘impossible’ molecule that could power quantum computers“. Additional details from an IBM Research press release “IBM & Warwick Image Highly Reactive Triangular Molecule for the First Time“:
Published today in Nature Nanotechnology, IBM scientists are truly making the invisible visible.
A few weeks ago IBM released its annual five predictions for the next five years based on this theme. IBM scientists in Zurich are making a good argument to add a sixth prediction with their latest scientific achievement – imaging some of the tiniest objects known to science.
While not household names, molecules including pentacene, olympicene, hexabenzocoronene and cephalandole A are all microscopic molecules which are traditionally represented using 2D structural stick models – think back to your high school chemistry class.
But thanks to a microscopy technique published by the IBM scientists in 2009, physicists, biologists and chemists around the world can now image these molecules with remarkable clarity and precision, in some cases for the first time, decades after they were first theorized allowing them to study and manipulate with incredible precision.
David Fox, University of Warwick, explains “For chemists it is amazing to be able to see individual molecules in such high resolution, especially unusual or highly reactive ones. It is the best way to confirm their structure.”
In addition to imaging, the IBM team, which includes two European Research Council (ERC) grant winners, Leo Gross and Gerhard Meyer [who are also two of the three-member IBM Research-Zurich team who won the 2012 Foresight Institute Feynman Prize for Nanotechnology for Experiment], is also able to manipulate molecules to cause chemical reactions so molecules can be synthesized from adsorbed precursor molecules.
For example, nearly one year ago in collaboration with CiQUS at the University of Santiago de Compostela, the scientists triggered and observed a fascinating molecular rearrangement reaction known as a Bergman cyclisation and the year before that they studied and visualized arynes, a family of highly-reactive short-lived molecules which was first suggested 115 years ago — proving that they do in fact exist. And now, they are doing it again.
Appearing today in Nature Nanotechnology [abstract], IBM scientists in collaboration with chemists at the University of Warwick have synthesized and characterized a tricky molecule called triangulene, also known as Clar’s hydrocarbon, which was first hypothesized in 1953.
Anish Mistry, University of Warwick continues, “Chemists have always thought that triangulene would be too unstable to isolate. Building on our previous olympicene collaboration, we have added an extra ring to the molecule, and an extra level of complexity to the science, but have managed to make a previously impossible molecule with potentially really interesting properties.”
First author on the paper, IBM researcher, Niko Pavliček comments, “In this work, we used our atomic manipulation technique from the aryne and Bergman papers to generate triangulene, which had never been synthesized before. It’s a challenging molecule because it’s highly reactive, but it’s also particularly interesting because of its magnetic properties.”
As they have demonstrated in previous papers, IBM scientists use a unique combined scanning tunneling microscope (STM) and atomic force microscope (AFM), both invented by former IBM scientists in the 1980s and recognized with the Nobel and Kavli Prizes, respectively.
In their latest research the sharp tip of the combined STM/AFM was used to remove two hydrogen atoms from the precursor molecule. The STM takes its measurement by quantum mechanical tunneling of electrons between a tip brought very close to a sample surface and applying a voltage between them. At appropriately high voltage, the ‘tunneling electrons’ can induce the removal of the specific bonds within the precursor molecule. The product molecule can then be characterized by its molecular orbitals when imaging at milder voltages.
These measurements, combined with density functional theory calculations, confirmed that triangulene keeps it free molecules’ properties on the surface.
The team also used the AFM, with a tip terminated with a single carbon monoxide molecule, to resolve or image the planar molecule with its six fused benzene rings, which appear in a symmetric triangle, for the first time. The results produced some pleasant surprises.
Gross explains, “Radicals feature unpaired electrons, and we previously were investigating sigma-radicals. In these, the unpaired electrons are assigned to certain atoms and we found that these always formed bonds with copper. But we were surprised that no bond formed for triangulene on copper. We think that is because triangulene is a pi-radical, which means it’s unpaired electrons are delocalized.”
It is exactly these unpaired electrons, which make the molecule interesting. In classical physics, a charged particle moving in space possesses angular momentum and produces a magnetic field around it. In quantum mechanics, every particle – moving in space or not – possesses an additional intrinsic angular momentum, which is called their ‘spin’. In most conventional hydrocarbons, electrons are always paired and the effect of their spins cancels. But in molecules like triangulene, the spin of the unpaired electrons leads to magnetism on the molecular scale.
The authors believe that beyond just the science there are also several interesting applications for this work.
Pavliček explains, “Triangulene-like segments incorporated into graphene nanoribbons have been suggested as an elegant way to design organic spintronic devices.”
Graphene nanoribbons are being researched for applications in nanocomposites materials, which are very strong and light. The field of spintronics is being studied by groups around the world, including at IBM, for information storage and processing.
Pavliček continues, “We could also demonstrate that its magnetism survives on xenon or sodium chloride surfaces. However, we cannot get a detailed picture of its magnetic state and possible excitations with our microscope (which lacks a magnetic field), so there is plenty to explore and discover for other groups.” …
This work is another impressive step forward by Leo Gross, Gerhard Meyer, and their IBM team members over the past dozen years imaging and manipulating individual chemical bonds using scanning probe microscopy, specifically an ultrastable combination STM/AFM with an ultrasharp tip terminating in a single carbon monoxide molecule. Previous milestones include:
- Imaging the individual chemical bonds in a pentacene molecule (“The Chemical Structure of a Molecule Resolved by Atomic Force Microscopy” 2009, abstract, PDF, Nanodot post)
- Measuring length and bond order of individual carbon-carbon bonds (“Bond-Order Discrimination by Atomic Force Microscopy” 2012, abstract, PDF requires free registration, Nanodot post)
- (“On-surface generation and imaging of arynes by atomic force microscopy” 2015, abstract, PDF, Chemistry World blog by Philip Ball)
- Rearranging chemical bonds on a surface (“Reversible Bergman cyclization by atomic manipulation” 2016, abstract, PDF, Chemistry World blog by Philip Ball)
Despite containing 22 carbon atoms and 12 hydrogen atoms arranged as six fused benzene rings, it is not possible to draw the familiar benzene resonant structures for the whole molecule without generating two unpaired valence electrons. The flat, triangular triangulene molecule is thus a diradical and very unstable and cannot be synthesized by conventional chemical methods. Several different isomers of dihydrotriangulene (22 carbon atoms and 14 hydrogen atoms) can be chemically synthesized, but these contain two or more tetrahedrally bonded methylene bridge carbon atoms instead of all flat aromatic carbon atoms. Dihydrotriangulene can be synthesized in a number of isomers—different molecules with the same number of each kind of atom, but joined together differently to form different structures.
The chemically synthesized dihydrotriangulene, consisting of mixtures of several structural isomers of dihydrotriangulene, was deposited in ultrahigh vacuum at 5 K on surface of either Cu(111), NaCl(100), or Xe(111). Imaging by STM and AFM revealed the presence of four isomers of dihydrotriangulene on the surface. The observed relative abundance of different isomers was consistent with energies calculated using density functional theory. By precise positioning of the tip over promising isomers, combined with careful control of current and voltage, followed by imaging, they were able to demonstrate the removal of single hydrogen atoms from methylene bridge CH2 groups. The voltage pulse was applied twice to individual dihydrotriangulene isomers to generate triangulene. Because the D3h symmetry of triangulene does not match the square lattice of (100)-oriented NaCl surfaces, the mismatch leads to frequent rotation between four equivalent adsorption geometries, the authors explain, so they chose further characterization of triangulene on Cu(111) and Xe(111) surfaces.
AFM results demonstrate stable adsorption of triangulene on Cu, with no indication of chemical bonding to the supporting Cu surface. This result contrasts with their previous observations of strong bonding to the Cu(111) surface of diradicals formed from arynes or Bergman cyclization products (above). They rationalize this discrepancy as due to the arynes and Bergman cyclization diradicals being σ radicals while the triangulene diradicals are π radicals. A comparison of STM and scanning tunneling spectroscopy results with density functional theory calculations indicate the properties expected of a triangulene diradical with two unpaired electrons having aligned spins.
A commentary in Nature by Philip Ball cites Philip Moriarty of the University of Nottingham, UK, describing the creation of triangulene as a new type of chemical synthesis in which atoms on individual molecules were physically manipulated using a microscope. In addition to the possible applications mentioned in spintronics and quantum computing, it will be interesting to see how far these recent discoveries can be extended building increasingly complex molecules, molecular machines, and molecular machine systems by assembling radicals on a surface. Perhaps a step on the path to general purpose, high throughput atomically precise manufacturing?
—James Lewis, PhD
Discuss these news stories on Foresight’s Facebook page or on our Facebook group.