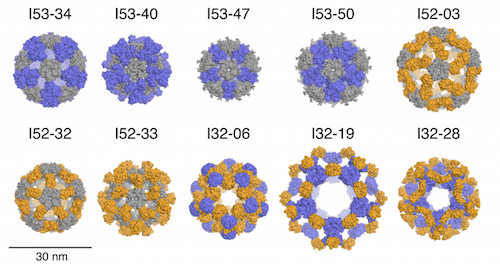
Baker Lab researchers have extended their work that we cited last summer assembling a large, stable, icosahedral protein molecular cage to a multi-component icosahedral protein complex. From a University of Washington Institute for Protein Design news release “Designed Protein Containers Push Bioengineering Boundaries“:
…Baker lab scientists and collaborators have taken this work to an exciting new level by engineering 120-subunit icosahedral nanocages that self-assemble from not one, but two distinct protein components. The new designed proteins are described in the latest issue of Science in a paper entitled “Accurate design of megadalton-scale multi-component icosahedral protein complexes” [abstract, full text PDF courtesy of Baker Lab].
In this paper, former Baker lab graduate student Jacob Bale, Ph.D. and collaborators describe the computational design and experimental characterization of ten two-component protein complexes that self-assemble into nanocages with atomic-level accuracy. These nanocages are the largest designed proteins to date with molecular weights of 1.8-2.8 megadaltons and diameters comparable to small viral capsids. The structures have been confirmed by X-ray crystallography (see figure). The advantage of a multi-component protein complex is the ability to control assembly by mixing individually prepared subunits. The authors show that in vitro mixing of the designed subunits occurs rapidly and enables controlled packaging of negatively charged GFP by introducing positive charges on the interior surfaces of the two components.
The ability to design, with atomic-level precision, these large protein nanostructures that can encapsulate biologically relevant cargo and that can be genetically modified with various functionalities opens up exciting new opportunities for targeted drug delivery and vaccine design. …
The above news release also links to a feature article, written by Robert F. Service, and video in Science “This protein designer aims to revolutionize medicines and materials” that provides an excellent overview and perspective on the work of David Baker, co-winner of the 2004 Feynman Prize, Theory category.
Citing their previous successes designing 24-subunit two-component tetrahedra, and noting the need for larger assemblies to package substantial amounts of cargo. Icosahedral symmetries generally enclose the maximum volumes, and the twofold, threefold, and fivefold rotational axes present within icosahedral symmetry provide three possible ways to construct complexes from pairwise combinations of oligomeric building blocks, designated I53, I52, and I32 architectural types. These three architectures are respectively formed from 12 pentameric and 20 trimeric building blocks, from 12 pentamers and 30 dimers, and from 20 trimers and 30 dimers.
From among several hundred thousand possible pairings of pentamers, trimers, and dimers, filtering based on a variety of metrics selected for experimental characterization 71 designs of type I53, 44 of I52, and 68 of type I32. These were derived from 23 pentameric, 57 trimeric, and 91 dimeric protein scaffolds. Experimental results are reported for two-component, 120-subunit icosahedral protein nanostructures of 1.8 to 2.8 megadalton molecular weight and 24 to 40 nm diameter. Ten designs spanning all three architectures form materials closely matching the design models.
The resolution obtained with x-ray crystal structures (0.35 to 0.56 nm) was not sufficient to determine side chain structures at the interfaces, the experimental structures matched the design structures with high accuracy at the level of polypeptide backbone structure. With more than 130,000 heavy atoms (all atoms except hydrogen) each, these are, to the best of the authors’ knowledge, the largest designed biomolecular nanostructures to date (July 2016) to be verified by x-ray crystallography.
The authors note that he designs presented here obey strict icosahedral symmetry, with the asymmetric unit in each case containing a heterodimer comprising one subunit from each of the two components. The authors are not aware of any natural protein complexes characterized to date that exhibit I52 or I32 architectures. “Our designs thus appear to occupy new regions of the protein assembly universe, which either have not yet been explored by natural evolution or are undiscovered at present in natural systems.”
The results presented here justify the authors’ conclusion in their abstract: “The ability to design megadalton-scale materials with atomic-level accuracy and controllable assembly opens the door to a new generation of genetically programmable protein-based molecular machines.” They suggest applications in areas like targeted drug delivery, vaccine design, and bioenergy. From the standpoint of productive nanosystems and atomically precise manufacturing, it will be interesting to see if such molecular machines can be adapted to the design of more complex and capable molecular machines, thus starting a virtuous cycle of tools producing better tools.
—James Lewis, PhD
Discuss these news stories on Foresight’s Facebook page or on our Facebook group.