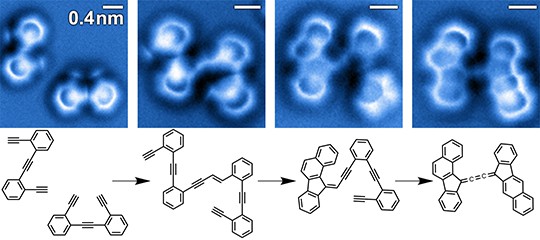
One path to advanced nanotechnologies begins with using scanning probe microscopes (SPM) to make atomically precise surface modifications—see, for example p. xii of Productive Nanosystems: A Technology Roadmap. The more precisely the SPM tip can image and manipulate atoms on a surface, the more rationally this path can be planned and implemented. Some of the most impressive progress along this path has come from using noncontact-atomic force microscopy (NC-AFM), such as measuring individual chemical bonds using a carbon monoxide-functionalized tip. Now researchers have succeeded in seeing changes in bond configurations as an organic reaction is catalyzed on a surface. A hat tip to Nanowerk for reprinting this press release from the Max Planck Institute for the Structure and Dynamics of Matter “Viewing a catalytic reaction in action“:
To be able to follow and directly visualize how the structure of molecules changes when they undergo complex chemical transformations has been a long-standing goal of chemistry. While reaction intermediates are particularly difficult to identify and characterize due to their short lifetimes, knowledge of the structure of these species can give valuable insights into reaction mechanisms and therefore impact fields beyond the chemical industry, such as materials science, nanotechnology, biology and medicine. Now an international team of researchers led by Felix R. Fischer, Michael F. Crommie (University of California at Berkeley and Lawrence Berkeley National Laboratory), and Angel Rubio (Max Planck Institute for the Structure and Dynamics of Matter at CFEL in Hamburg and University of the Basque Country in San Sebastián) has imaged and resolved the bond configuration of reactants, intermediates and final products of a complex and technologically relevant organic surface reaction at the single-molecule level. The findings are published in the journal Nature Chemistry [abstract].
Chemical transformations at the interface between solid/liquid or solid/gaseous phases of matter lie at the heart of key industrial-scale manufacturing processes. Understanding the microscopic mechanisms of such surface-catalyzed organic reactions is a grand challenge for modern heterogeneous catalysis and its application to industrial-scale chemical processes. Competing pathways that lead to numerous intermediates and undesired side products often hamper investigation of the underlying reaction mechanisms of reactions in chemical technology, such as the transformation of crude feedstock into complex value-added chemicals at the surface of a heterogeneous catalyst bed. The precise structural identification of transient reaction intermediates can be particularly difficult due to their low concentrations in the sample stream.
In the present work, the chemical structures associated with different steps of a reaction cascade of enediyne molecules on a silver surface were imaged using noncontact atomic force microscopy (nc-AFM) with special functionalized tips (using a carbon monoxide molecule to enhance resolution). Identification of the precise bond configuration of the intermediate species has allowed determining the sequence of chemical transformations along the pathway from reactants via intermediates to end products and unraveling the microscopic mechanisms behind that intricate dynamical behavior. “It was striking to be able to directly measure and theoretically characterize the chemical structure of reaction intermediates in this complex system,” said Felix Fischer, Professor for Chemistry at the University of Berkeley California and co-lead author of the study.
“This is a huge step for chemical synthesis,” added co-lead author Angel Rubio, Director at the Max Planck Institute for the Structure and Dynamics of Matter in Hamburg and Distinguished Professor for Physics at the University of the Basque Country. “However, we wanted to go deeper and understand why the intermediates are stabilized on the surface – this does not happen in solution.” A combination of extensive state-of-the-art numerical calculations with classic analytical models describing the kinetics of sequential chemical reactions has shown that it is not enough to consider the energy potential landscape (i.e. the energies of the species along the reaction pathway and the associated transformation barriers), but that energy dissipation to the substrate and changes in molecular entropy play a critical role for the stabilization of the intermediates. The surface, and in particular the interaction of molecular radicals with the surface, plays a key role for both, entropy and selective dissipation, highlighting fundamental differences of surface-supported reactions compared to gas-phase or solution chemistry.
“The fruitful collaboration between theory and experiment allowed us to identify the microscopic driving forces that govern the global reaction kinetics,” said Alexander Riss, first author of the study. Such detailed understanding constitutes a fundamental milestone in the analysis of chemical reactions that was achieved through the synergy between single-molecule visualization of chemical reactions and state-of-the-art high-performance computer modeling. By these means, many limitations of conventional ensemble averaging spectroscopic techniques are surpassed, and an unprecedented atomic-scale picture of the reaction mechanisms, driving forces and kinetics emerges. Such new insight may open countless of hitherto unexplored venues for the future design and optimization of heterogeneous catalytic systems, for the development of novel synthetic tools applied to carbon-based nanotechnology, as well as for biochemical and materials science applications.
The immediate and near-term application of this research is no doubt the design of better catalysts, but these methods may also speed the use of scanning probe tips to build increasingly complex atomic configurations on surfaces.
—James Lewis, PhD
Discuss these news stories on Foresight’s Facebook page or on our Facebook group.